正規分布の母平均μに対してθ=exp(μ)をパラメータとする最尤推定量のバイアスを調べて、与式がθの不偏推定量であることを示しました。
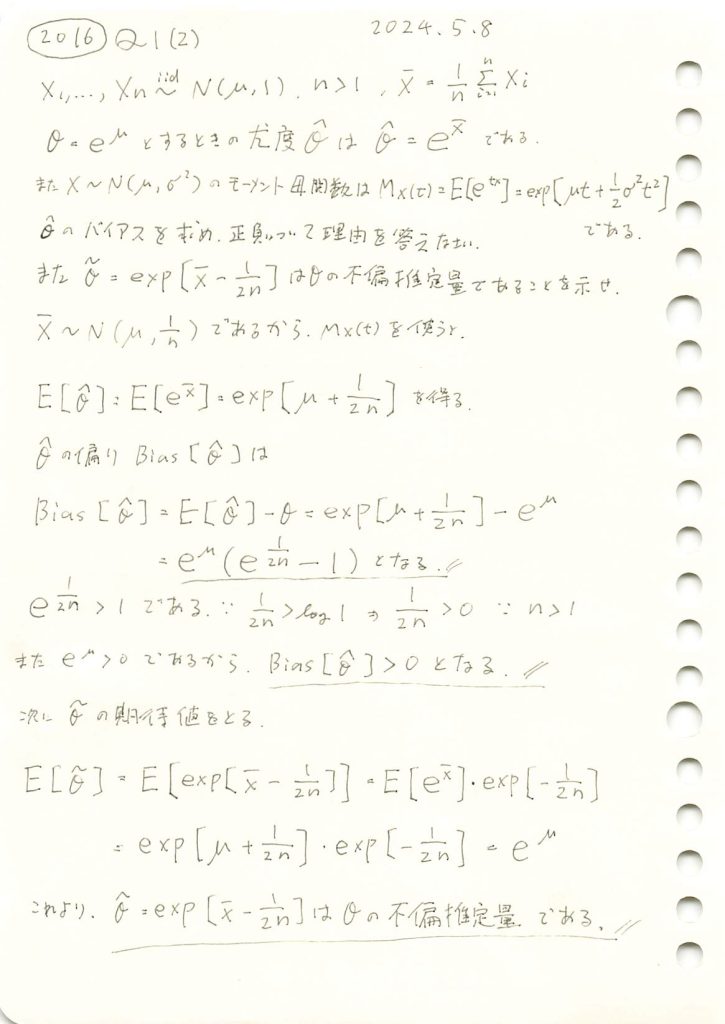
コード
と
が真のθに近づくか確認するため、シミュレーションを行います。まずはサンプルサイズn=5で試してみます。
# 2016 Q1(2) 2024.11.12
import numpy as np
import matplotlib.pyplot as plt
# シミュレーションのパラメータ
mu_true = 1.0 # 真の平均 (mu)
theta_true = np.exp(mu_true) # 真の θ = e^mu
n_samples = 5 # サンプルサイズ
n_trials = 100000 # シミュレーション試行回数
# 乱数生成と推定量の計算
theta_hat_estimates = []
theta_tilde_estimates = []
for _ in range(n_trials):
sample = np.random.normal(loc=mu_true, scale=1/np.sqrt(n_samples), size=n_samples)
X_bar = np.mean(sample) # 標本平均
# 最尤推定量 θ^
theta_hat = np.exp(X_bar)
theta_hat_estimates.append(theta_hat)
# 不偏推定量 θ~
theta_tilde = np.exp(X_bar - 1/(2*n_samples))
theta_tilde_estimates.append(theta_tilde)
# 推定量の平均を計算
theta_hat_mean = np.mean(theta_hat_estimates)
theta_tilde_mean = np.mean(theta_tilde_estimates)
# グラフ描画
plt.figure(figsize=(12, 6))
# ヒストグラムの描画
plt.hist(theta_hat_estimates, bins=100, density=True, alpha=0.6, color='blue', label='$\\hat{\\theta}$ (最尤推定量)')
plt.hist(theta_tilde_estimates, bins=100, density=True, alpha=0.6, color='green', label='$\\tilde{\\theta}$ (不偏推定量)')
# 真の値を赤線で表示
plt.axvline(theta_true, color='red', linestyle='dashed', linewidth=2, label=f'真の$\\theta$: {theta_true:.2f}')
# θハットとθチルダの平均をそれぞれ緑線と青線で表示
plt.axvline(theta_hat_mean, color='blue', linestyle='dotted', linewidth=2, label=f'$\\hat{{\\theta}}$平均: {theta_hat_mean:.2f}')
plt.axvline(theta_tilde_mean, color='green', linestyle='dotted', linewidth=2, label=f'$\\tilde{{\\theta}}$平均: {theta_tilde_mean:.2f}')
# グラフのタイトルとラベル
plt.title('$\\hat{\\theta}$ と $\\tilde{\\theta}$ の分布', fontsize=16)
plt.xlabel('$\\theta$', fontsize=14)
plt.ylabel('密度', fontsize=14)
plt.legend(fontsize=12)
plt.grid(True)
plt.show()
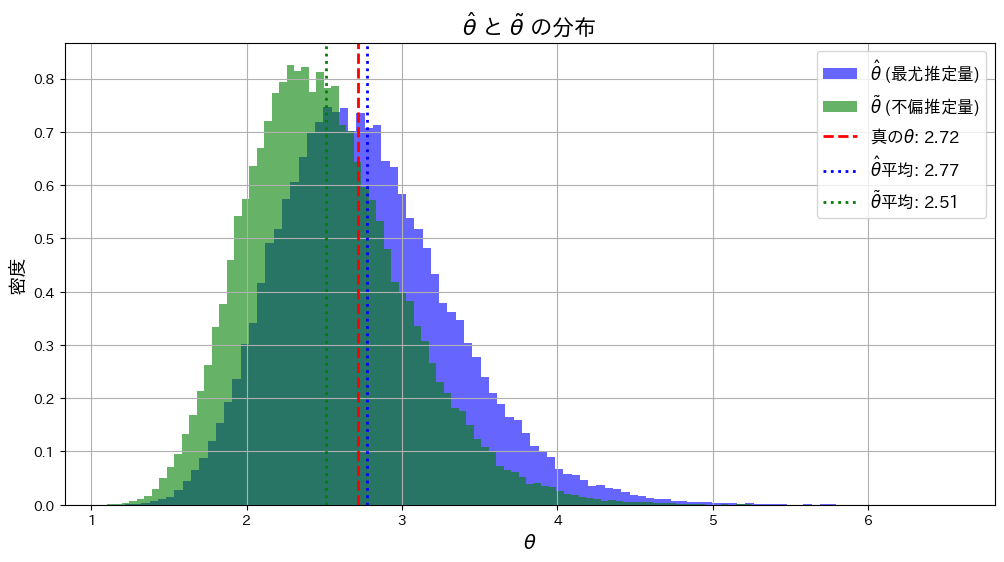
は
よりも真のθに近くなっています。これはサンプルサイズnが小さいことと、および推定量の分散の違いが起因しているのかもしれません。
次に、サンプルサイズn=5, 10, 20, 50と変化させて、この乖離がどのように変わるかを確認します。
# 2016 Q1(2) 2024.11.12
import numpy as np
import matplotlib.pyplot as plt
# シミュレーションのパラメータ
mu_true = 1.0 # 真の平均 (mu)
theta_true = np.exp(mu_true) # 真の theta = e^mu
n_trials = 100000 # シミュレーション試行回数
sample_sizes = [5, 10, 20, 50] # サンプルサイズのリスト
fig, axes = plt.subplots(2, 2, figsize=(12, 10))
axes = axes.ravel() # 2x2 の配列を平坦化
for i, n_samples in enumerate(sample_sizes):
theta_hat_estimates = []
theta_tilde_estimates = []
for _ in range(n_trials):
sample = np.random.normal(loc=mu_true, scale=1/np.sqrt(n_samples), size=n_samples)
X_bar = np.mean(sample)
# 最尤推定量
theta_hat = np.exp(X_bar)
theta_hat_estimates.append(theta_hat)
# 不偏推定量
theta_tilde = np.exp(X_bar - 1/(2*n_samples))
theta_tilde_estimates.append(theta_tilde)
theta_hat_mean = np.mean(theta_hat_estimates)
theta_tilde_mean = np.mean(theta_tilde_estimates)
# ヒストグラムのプロット
axes[i].hist(theta_hat_estimates, bins=100, density=True, alpha=0.6, color='blue', label='$\\hat{\\theta}$ (最尤推定量)')
axes[i].hist(theta_tilde_estimates, bins=100, density=True, alpha=0.6, color='green', label='$\\tilde{\\theta}$ (不偏推定量)')
axes[i].axvline(theta_true, color='red', linestyle='dashed', linewidth=2, label=f'真の$\\theta$: {theta_true:.2f}')
axes[i].axvline(theta_hat_mean, color='blue', linestyle='dotted', linewidth=2, label=f'$\\hat{{\\theta}}$平均: {theta_hat_mean:.2f}')
axes[i].axvline(theta_tilde_mean, color='green', linestyle='dotted', linewidth=2, label=f'$\\tilde{{\\theta}}$平均: {theta_tilde_mean:.2f}')
axes[i].set_title(f'サンプルサイズ n={n_samples}')
axes[i].set_xlabel('$\\theta$')
axes[i].set_ylabel('密度')
axes[i].legend()
axes[i].grid(True)
plt.suptitle('$\\hat{\\theta}$ と $\\tilde{\\theta}$ の分布', fontsize=16)
plt.tight_layout(rect=[0, 0, 1, 0.95])
plt.show()
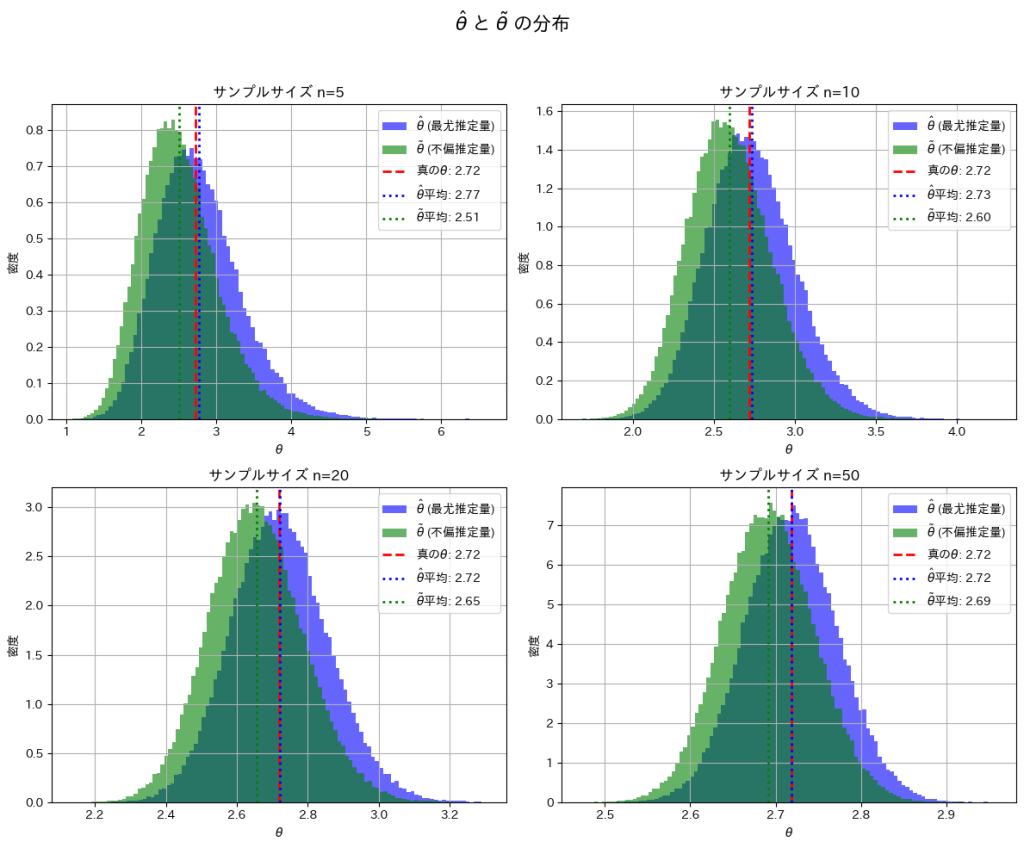
nを大きくすることで、と
は真のθの間の乖離が小さくなることを確認できました。
次に、nを変化させてと
がどのように変化するか折れ線グラフで見てみます。
# 2016 Q1(2) 2024.11.12
import numpy as np
import matplotlib.pyplot as plt
# シミュレーションのパラメータ
mu_true = 1.0 # 真の平均 (mu)
theta_true = np.exp(mu_true) # 真の theta = e^mu
n_trials = 100000 # シミュレーション試行回数
sample_sizes = np.arange(5, 101, 5) # サンプルサイズ n の範囲 [5, 10, ..., 100]
# 推定量の平均値を格納するリスト
theta_hat_means = []
theta_tilde_means = []
# サンプルサイズを変化させてシミュレーション
for n_samples in sample_sizes:
theta_hat_estimates = []
theta_tilde_estimates = []
for _ in range(n_trials):
sample = np.random.normal(loc=mu_true, scale=1/np.sqrt(n_samples), size=n_samples)
X_bar = np.mean(sample)
# 最尤推定量
theta_hat = np.exp(X_bar)
theta_hat_estimates.append(theta_hat)
# 不偏推定量
theta_tilde = np.exp(X_bar - 1/(2*n_samples))
theta_tilde_estimates.append(theta_tilde)
# 平均値を格納
theta_hat_means.append(np.mean(theta_hat_estimates))
theta_tilde_means.append(np.mean(theta_tilde_estimates))
# 折れ線グラフの描画
plt.figure(figsize=(10, 6))
plt.plot(sample_sizes, theta_hat_means, marker='o', linestyle='-', color='blue', label='$\\hat{\\theta}$ (最尤推定量)')
plt.plot(sample_sizes, theta_tilde_means, marker='s', linestyle='-', color='green', label='$\\tilde{\\theta}$ (不偏推定量)')
plt.axhline(y=theta_true, color='red', linestyle='dashed', linewidth=2, label=f'真の$\\theta$: {theta_true:.2f}')
# ラベルとタイトル
plt.title('サンプルサイズ n に対する $\\hat{\\theta}$ と $\\tilde{\\theta}$ の平均値', fontsize=16)
plt.xlabel('サンプルサイズ $n$', fontsize=14)
plt.ylabel('$\\theta$の平均値', fontsize=14)
plt.legend(fontsize=12)
plt.grid(True)
plt.show()
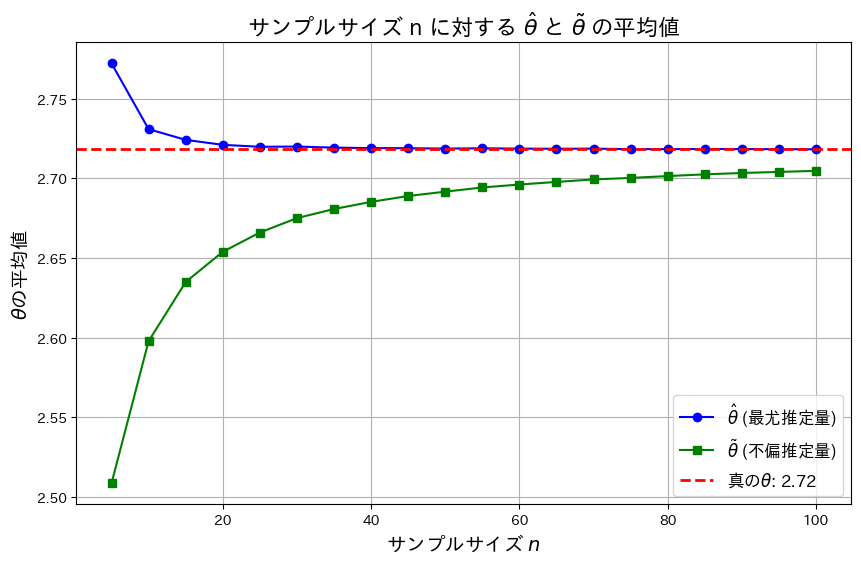
は真のθよりも大きい値を取り、徐々に減少し真のθに近づきます。一方
真のθよりも小さい値を取り、徐々に増加し真のθに近づきます。また近づくスピードは
のほうが早く、
の分散が
の分散よりも小さいことが分かります。